
Why Does A Tyre Grip The Road? Part 2 - Grip
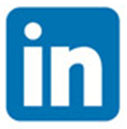
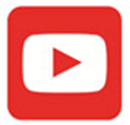
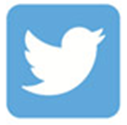
Slippage
Coloumb's Friction model is a simple but accurate model for calculating the magnitude and direction of a friction force. For dry static friction, the model simply states that the magnitude of friction is directly proportional to the vertical force (Z) being placed upon it and the coefficient of static friction (μs), which as we see a bit later on depends on a number of factors. The direction of the friction force will be opposite that of the external force and will equal the external force up to the coefficient of friction's limit. After this point we are in kinetic friction territory. As you can imagine Coloumb studied this as well and came up with a model for kinetic friction. Lucky for us we simply swap out the coefficient of static friction for one of kinetic friction (μk).

As you have probably observed, to move something that is currently at rest is far harder than continuing to move something, the reason for this is that kinetic friction is lower than that of static friction. Static friction is able to create a strong bond between a block and the ground, whereas a moving block has less time and therefore the bond isn't as strong.
There are three main rules for Columb's friction model are
- The frictional force is proportional to the normal force.
- The frictional force is independent of the apparent area of contact.
- The frictional force is independent of the relative velocity.
Below is a picture of what the transition from shear to slippage looks like for a rubber block. Once the force is so great that the coefficient of static friction is exceeded, the block brakes away and begins to move. We are in the kinetic region, with a maximum grip force below that of the static maximum.


R = Rolling radius, combined (⍵R) are the rolling speed of the tyre, and
V = The vehicle’s speed.
Rough road effect
If a section of road is zoomed in on, the viewer would see tiny hills and valleys. As the tyre travels over these, the rubber deforms, it flows over the hills and fills the valleys like a viscous fluid. As the tyre flows over the top of the hill and begins down the back side, it starts to relax, morphing back into its original shape. The gradual change, due to the viscous properties of the rubber means that some of the energy is lost from the system. As we saw in the first post, this loss comes from the difference between rubbers loading and unloading stress strain curves.
If we look at figure 5 and imagine the contact patch is moving to the right, as the rubber impacts the hill and absorbs the energy, this is done quite quickly. But as it comes back down the other side we can see that it takes some time to get back to its relaxed state, some of the energy is lost, meaning that there is now a higher force on the left side of the hill than the right. This force imbalance results in a leftward facing force, X in the image, that opposes the slippage.
Molecular Adhesion
Whilst the road roughness effect relies on hills and valleys in the road, molecular adhesion needs smooth surfaces to work; it relies on direct contact between the road and the tyre. As the polymer chain comes into contact with the road they stick to one another, they are able to do this through a phenomenon called Van der Waals bonding. As the contact patch continues along, the polymer, still holding on, stretches until the bond is broken, it then relaxes. This stick, stretch, relax process repeats until that polymer strand is no longer in the contact patch. As stated in the last article, polymer strands can be thought of as springs, as they stretch they store energy. This energy is then released as the polymer chains detach and relax, however only part of the energy is recovered. The strands continue to rub against themselves and each other creating internal friction. This friction results in lost energy, it creates the tangential friction force, it creates grip.
Road roughness' impact on grip
A road's roughness impacts what percentage of each of the two grip mechanisms are being used at any given point in time. Road roughness obviously needs hills and valleys to work whereas molecular adhesion needs flat surfaces, but there is a range where each works at its optimum. For road roughness the optimum peak hill distance (D in figure 5 above) is in the macroroughness range, that is between 0.1 - 10mm. Molecular adhesion on the other hand works best in the microroughness range, that is between 0.001 - 0.1mm.
Water's impact on grip
As you know a road’s roughness isn't the only thing that impacts grip, so does its wetness. Water on the road forms a film that lays between the tyre and the road, this film blocks molecular adhesion, rendering it useless (see figure 7). Whilst molecular adhesion doesn’t work, depending on the depth of the valleys and the level of water on the road, the tyre is still able to deform as it travels over the hills, so the road roughness effect continues to work. A good rule of thumb is that grip will continue to be there for water levels up to about 1mm above the road surface, after this point a tyre will typically begin to hydroplane (aquaplane).
Whilst the depth of water has a massive impact on the level of grip, it isn’t the only factor, speed, tyre tread patterns, road surface, tyre wear, road wear, and tyre make up all play a part. The graph below shows the impact of speed, water depth, and level of tyre wear on grip. Interestingly, at low speeds grip is fairly stable despite increasing levels of water, whilst at high speeds, grip is heavily affected by the water depth. We can also see that the gap between the good tyre and the worn tyre (half skid) at higher speeds is far greater than that at lower speeds.
Another interesting study done by Beautru, Y. 2011, shows that road roughness also has a dramatic impact on the level of grip in the wet. Below we can see that for the smoother road, Mosaic, it initially has a higher coefficient of friction, but reduces far more dramatically then the rougher road, Aged VTAC, with increasing levels of water. If we divide the plots into three groups, Dry, Mixed, and Hydrodynamic we can see some interesting things. The first is that there is two stable friction levels, one in the dry region and another in the hydrodynamic region. The Mosaic, despite initially having higher levels of grip in the dry quickly stabilisers at the same friction value as the rougher road, but then falls off a clip and re-stabilsers at a much lower value in the hydrodynamic zone. The re-stabilisation within the hydrodynamic region is very interesting, its not something that I would have thought occurred. The sharpness of Mosaic's mixed zone, the early onset of it, and the lower hydrodynamic value are all due to there being no valley's for the water to fill up creating a thicker broundary layer between the road and the tyre. An increase of roughly 0.175mm of water for the Mosaic road reduces the friction coefficient from an average dry road value to its hydrodynamic figure, which offers just 40% of the dry roads grip, crazy stuff.

As you have no doubt experienced, predicting the weather on a race day and therefore what tyres and pressures to choose is already quite difficult. If the track is especially smooth, this can be made even more challenging and the results of which more dramatic.
This takes us to the end of another post, but never fear, we have other great posts already up for you to enjoy and will continue to post into the future. Next we will be looking into longitudinal grip, the link for it is below, but if you want to check out something else tyre related, see the links to the series below. Don’t forget to sign up so you don’t miss an article and as always, if you have any comments, suggestions, or noticed I made a mistake, either write it down in the comments or get in contact via email or using the contact page, I’d love to hear it. Thanks for reading and I hope you have a great day.
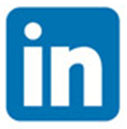
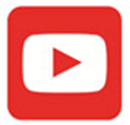
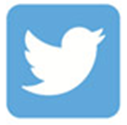
Why Does A Tyre Grip The Road Series
References:
[1] nextgen-auto motorsport, 2022, https://motorsport.nextgen-auto.com/fr/formule-1/photos/photos-la-mclaren-f1-mcl36-en-piste-a-barcelone,168327.html, retrieved on 10/03/22[4] Chemistry Learner, Van der Waals Forces, Link, retrieved on 3/03/22
[5] Balkwill, J. 2007, Performance Vehicle Dynamics
[6] Kulakowski BT and Harwood DW. 1990, Effect of water-film thickness on tire-pavement friction. In: WE Meyer and J Reichter (eds) Surface characteristics of roadways: international research and technologies, ASTM STP 1031, pp.50–60. Philadelphia, PA: ASTM
[7] Beautru, Y.; Kane, M.; Cerezo, V.; Do, M.-T. Effect of thin water film on tire/road friction. In Proceedings of the Young ResearchersSeminar (YRS 2011), Copenhagen, Denmark, 8–10 June 2011
No comments:
Post a Comment